Fuelling the debate on diesel engine emissions
Posted: 28 May 2008 | | No comments yet
The International Union of Railways (UIC) was founded in 1922 to create standards to facilitate the operation of international railway traffic. These standards are contained within UIC-Leaflets which define technical specifications for interoperability for rolling stock, infrastructure, signalling systems and catenary. Other Leaflets cover agreements on operational and commercial rules. Some Leaflets are mandatory (e.g. braking systems, wheelsets, draw hooks and buffers) whereas others are only recommendatory.
The International Union of Railways (UIC) was founded in 1922 to create standards to facilitate the operation of international railway traffic. These standards are contained within UIC-Leaflets which define technical specifications for interoperability for rolling stock, infrastructure, signalling systems and catenary. Other Leaflets cover agreements on operational and commercial rules. Some Leaflets are mandatory (e.g. braking systems, wheelsets, draw hooks and buffers) whereas others are only recommendatory.
The International Union of Railways (UIC) was founded in 1922 to create standards to facilitate the operation of international railway traffic. These standards are contained within UIC-Leaflets which define technical specifications for interoperability for rolling stock, infrastructure, signalling systems and catenary. Other Leaflets cover agreements on operational and commercial rules. Some Leaflets are mandatory (e.g. braking systems, wheelsets, draw hooks and buffers) whereas others are only recommendatory.
At the beginning of the 1950s, the UIC member railways expressed a common interest in carrying out international research on diesel traction, primarily to address maintenance and traction problems. As a result, the daughter organisation of the UIC responsible for research, ORE, later to become ERRI, established an expert committee on a permanent basis to cover aspects relating to diesel traction.
Over the next 50 years, most European countries opted for wide-scale electrification programmes to replace steam, with very little use of diesel traction. In consequence, approximately 80% of the railway traffic in Europe travels over electrified lines.
Diesel emission standards
From the beginning of the 1990s, European railways have come under increasing pressure from the public perception of emission problems with their diesel locomotives. Up until 2004, limit values for exhaust emissions from railway diesel vehicles were controlled only by the applicable regulations of the UIC leaflets 623 and 624. Leaflet 623, introduced in 1982, describes the entire approval process for a diesel engine for use as a railway motive power unit. From its very first edition, critical aspects such as limit values for emissions of carbon monoxide (CO), unsaturated hydrocarbons (HC), nitrogen oxide (NOx) and exhaust blackness (Bosch number) were covered. However, leaflet 623 was only recommendatory, and was not applied by all UIC railways. Given the increasing sensitivity to the exhaust emissions produced by diesel vehicles, leaflet 624, mandatory for all member railways, was introduced in 2001. This separated exhaust emission control from the purely engine-specific approval procedure. A limit value for particle emissions was introduced which replaced the exhaust blacking (Bosch value) and an incremental reduction in the limit values (UIC I, UIC II) was stipulated, as well as differentiation between limit values for engines up to 560 kW from those with higher power.
In parallel with these developments, the European Commission began to introduce legislation limiting pollutant emissions for all Non Road Mobile Machinery (NRMM). EC Directive 97/68, in force since 1998, excluded rail vehicles. From the end of 1990s, a number of railways had been coming under the scrutiny of environmentalists since a growing source of complaints were being made concerning diesel emissions from rail vehicles in densely populated urban areas.
As a result, the revision (26/2004) of the EU directive 97/68, included provisions for railway vehicles in the directive and from this point on they became subject to statutory provisions regarding their exhaust emissions. Emission limits of different values were introduced for locomotive engines and for railcar engines (IIIA) between 2006 and 2009 and even stricter limits (IIIB) were envisaged for 1 January 2012. Simultaneously, it was decided to perform an inventory on emissions by Rail vehicles (Rail Diesel Study), an investigation of the validity of the rail specific test cycle (cycle F in ISO 8178-4) as well as a critical review of the new version of the Directive and their future limits to be completed by the end of 2007.
The inventory was performed by AEAT with support from the UIC, Rolling Stock Manufacturers (UNIFE) and Engine Manufacturers (EUROMOT). The resulting Rail Diesel Study was published in early 2006. The average age of the European Diesel fleet was estimated to be 27 years for locomotives and 16 years for DMUs. The rail sector’s total pollutant emission output was evaluated to be less than 1% of the total emission release. With an increasing replacement of existing rolling stock by new vehicles, a gradual improvement of the emission behaviour of the fleet was anticipated in the mid-term. Retrofitting the existing fleet, which in some cases was technically not feasible, was not considered to bring a net benefit.
The Review Process of the Directive started in 2006 under the responsibility of the European Commission’s Joint Research Centre (JRC).
To represent the opinion and needs of operators and manufacturers, the UIC B 208 expert committee, together with Rolling Stock Manufacturers (UNIFE) and engine producers (EUROMOT) was asked to define the users’ standpoint. The following work stages were identified:
- Assessment of modifications within the engine that might lead to potential improvement of its pollutant emissions
- Assessment of exhaust after-treatment systems and filters for railway operations together with their impact on fitment space in the vehicle, mass and length
- Consequences for re-engining
- Consequences for procurement, operational and maintenance costs
- Test cycle assessment
- Comparison and possible alignment with North American legislation
NOx reduction
Significant NOx-reduction is achievable by implementation of the so-called ‘Miller-cycle’, originally devised to improve the efficiency of SI-engines (spark ignited engines), for diesel engines. Instead of closing the inlet valve after the piston passed Bottom Dead Centre (BDC) on its intake stroke, the valve closes long before BDC. This allows an expansion of the charge air leading to a lower combustion temperature. The formation of NOx increases with the combustion temperature.
There are basically two methods of applying the Miller process. The so-called ‘early’ version, where the intake valve closes before BDC (500 – 540°), or the ‘late’ version, where the intake valve closes much later than BDC. Both methods lead to a reduction in effective compression. In the ‘early’ configuration, this results from expansion of the charge up to BDC and then a compression starting from a lower level of pressure and temperature, and in the ‘late’ configuration it results from the late closure of the intake valve at a time when the piston is already moving upwards once again.
Both procedures can be applied to diesel engines, where they reduce the final compression temperatures and produce lower peak temperatures during combustion.
A second method to reduce NOx by internal engine design is EGR. EGR works by re-circulating a portion of an engine’s exhaust gas back to the engine cylinders. Intermixing the incoming air with re-circulated exhaust gas dilutes the mix with inert gas, lowering the adiabatic flame temperature and (in diesel engines) reducing the amount of excess oxygen. The exhaust gas also increases the specific heat capacity of the mix, lowering the peak combustion temperature. Because NOx formation progresses much faster at high temperatures, EGR limits the generation of NOx.
Whereas the Miller-cycle would allow compliance with IIIA limits without any additional after-treatment, the trade-off on fuel consumption would be rather prohibitive when applied to ensure compliance with IIIB. EGR would allow IIIB limits to be achieved, but it requires a much more sophisticated cylinder head design with potential disadvantages in maintenance costs. There are other trade-offs. Adding EGR to diesel reduces the specific heat ratio of the combustion gases in the power stroke. This reduces the amount of power that can be extracted by the piston. EGR also tends to reduce the amount of fuel burned in the power stroke. This is evident by the increase in particulate emissions that correspond to an increase in EGR. In modern diesel engines, the EGR gas is cooled through a heat exchanger to allow the introduction of a greater mass of re-circulated gas. A schematic of an EGR system is given in Figure 2.
As both methods lead to a significant decrease in combustion temperature, they will not be able to lower particulate emissions since particulates are burnt off at higher temperatures. To comply with IIIB PM (particulate matter) limit values a particulate filter (DPF) becomes necessary.
SCR systems
Current exhaust after-treatment systems are not specifically geared to railway applications since the rail market is relatively small. Therefore, other existing after-treatment systems have to be considered and their suitability for use on railways assessed. The complete lack of operational experience, together with the potential risk of increasing maintenance costs, makes operators hesitate to introduce these systems. NOx-reduction by SCR (Selective Catalytic Reduction) converters requires urea to be used as an additional operational fluid. In this application, urea consumption is approximately 5% of the fuel consumption. Vehicles have to be equipped with a second tank for the urea, which must be protected from freezing (urea freezes at -11°C but starts to crystallise at around +5°C); urea is very aggressive so storage tanks and fuel lines have to be made from stainless steel. In addition, urea is not chemically stable, which necessitates additional logistics to avoid excessive storage periods.
Another potential disadvantage of SCR devices is their poor efficiency when used in railway applications. Idling times are long relative to working cycles. The resulting low exhaust gas temperatures mean that an SCR device will only function properly for 15% of the total useful lifetime of the engine. Side effects, such as ammonia slip, will cause additional problems.
A little-noted aspect of using urea selective catalytic reduction to control of NOx emissions from diesel engines is its effect on CO2 emissions. Optimal selective catalytic reduction operation requires approximately one mole of ammonia for every mole of nitrogen oxides reduced. In terms of mass, for a reduction of 1,000 kilograms of NOx, 479 kilograms of CO2 are released.
Last, but not least, the application of SCR devices would generate a noticeable increase in costs for the railways. An ATOC (Association of Train Operating Companies) study has revealed that in Great Britain, approximately 100 urea filling stations would be needed. This would result in an annual capital cost of between €15 to 18 million on the operators’ bills. Costs of similar magnitude for other Railways are to be envisaged.
Particulates
Capturing particulates in a DPF device results in a progressive plugging of the filter, with a consequent increase in the back pressure imposed on the engine. To reduce back pressure, the filter must be regenerated by burning off the soot and soluble organic fraction of the trapped particulates. Particulate filters are manufactured either with a catalytic coating or without a catalyst. The effect of a catalyst is to lower the temperature at which the captured carbon particles will burn off. Without a catalytic coating, it is necessary to raise the temperature of the filter to 600°C to burn off the carbon. Due to the relatively low exhaust temperatures of engines in railway operation, it seems certain that some form of active regeneration, either by electrically heating the filter or by burning additional fuel in the exhaust, will be necessary for regeneration. Of these two options, the more efficient, in terms of energy consumption, is burning fuel in the exhaust stream, but – in general – regeneration means extra energy consumption.
Application in railway service
As railway vehicles have stringent limitation on mass (an 18t axle load for secondary lines) as well as on fitment space, the introduction of external after-treatment devices such as SCR and/or DPF is of major concern for manufacturers and operators. Realistic assumptions show a weight increase of 3 to 4 tonnes requiring extra space of about 2m3 for a 4-axle main line locomotive with a 2000 kW engine and about 0,5-1 tonne for railcars when adding SCR+DPF.
The latest European rolling stock manufacturers’ investigations indicate that voluminous additional equipment will have to be installed in rail vehicles in order that they meet the new 2004/26/EC Stage IIIB emission limits. Not only does the space requirement of the after-treatment devices need to be considered, but also that for the increased auxiliary equipment such as heat exchangers, cooling fans and additional piping.
For DMUs, a potential loss of passenger capacity is to be anticipated, together with functional problems. If the position where after-treatment device is located (e.g. on the roof) is far away from the engine (often underfloor), this will result in undesirable cooling of the exhaust gases before they reach the reactor, leading to very poor efficiency.
The legislative dilemma
The service life of railway vehicles, whether locomotives or multiple-units, is much longer than in the road sector. As a result, rail operators tend to replace diesel engines halfway through the service life of the vehicle.
An additional problem caused by the Directive 26/2004 is the requirement that such replacement engines have to comply with current emission limits. At present, it is still fairly straightforward to replace an older engine by a new generation engine.
This is likely to change with the introduction of IIIB limits. The limits will necessitate additional space for after-treatment systems and bigger cooling equipment which will not fit into the vehicle. Fuel costs contribute to almost 90% of the Life Cycle Costs of the engine; the remaining 7% are maintenance costs. It is quite evident that new-for-old engine replacement will be paid off quickly purely through fuel savings.
Future IIIB compliant engines are unlikely to be more fuel efficient than UIC II or IIIA compliant engines. At some stage in a vehicle’s life, maintenance requirements would demand the replacement of the installed engine by a new type. If additional devices like DPF and SCR have to be fitted, an increase in service costs would be anticipated without a potential gain in fuel saving. In this case, replacement will not take place even if it is technically feasible. If a new engine gives emission benefits but at the same time only results in operational disadvantages, the owner is more likely to extend the life of the existing engine until the end of the useful life of the locomotive.
There is a risk that the requirements of the Directive, by their rigid nature, could backfire in the longer term, by keeping older engines in service rather than encouraging their replacement with more efficient ones. Some fairly simple adaptations to the Directive, along the lines of existing United States’ legislation, would offer greater flexibility and give railway operators an incentive to renew their motive power fleets more frequently.
Suitability of test cycles
Currently two different test cycles are to be applied to determine the pollutant emissions of the railway vehicle engines. For locomotive engines, the railway-specific F cycle of ISO 8178-4 is to be used, whereas the C1 cycle is imposed by EU 26/2004 Directive for DMU engines. With the introduction of the Directive, the EC asked for verification of the validity of the latter test cycle.
UIC report B 208/RP 6 (published in November 2006) considers the load spectra from eight European railways and reproduces the load spectra of around 1 million operating hours.
Rail vehicles of all types are represented, from DMUs through main-line locomotives for passenger services and shunting locomotives to heavy freight locomotives.
The current F cycle of ISO 8178-4 is a steady state three-mode cycle with 25% rated speed, 15% intermediate load and 60% idling. It is evident that, on average, this cycle is the most appropriate for the various railway application cases; however the weighting factors for full load and partial load need to be adjusted.
The idling portion is on average almost identical to that in the current ISO 8178-4 F cycle.
The high idling portion of railway vehicles is due to their low running resistance (approximately 10% of a road vehicle’s running resistance). After an initial acceleration phase, with high load applied immediately after train start, a small load is sufficient to keep the train coasting at a given speed on a level track, and to produce sufficient energy for auxiliary drives. Therefore it happens that sometimes mainline locomotives, which are never used for shunting purposes, have idling percentages which are not significantly lower than those of other vehicles.
A comparison of the results with the C1 cycle imposed for DMU engines clearly demonstrates that this test cycle is not at all appropriate for railway engines and a distinction between DMU engines and locomotive engines cannot be justified.
The survey proved that, firstly, cycle F of the ISO 8178-4 represents the best compromise as a test cycle for railway vehicles, and, secondly, that a differentiation between locomotives and motorised trains is not justified for the application of the test cycle.
Although the engines currently in use in the rail vehicle sector have not been specially developed for railway applications (with the exception of the large US locomotive engines which have a very small market share in continental Europe) they are, however, optimised by the manufacturer for use in the railway sector. This includes adjustment to the currently valid test cycle. When engines are optimised for a test cycle that does not correspond at all with the actual operational conditions, there is a potential risk that such engines will exhibit significantly poorer exhaust behaviour in actual operational use.
The regulatory framework needs to consider the characteristics of a niche market for NRMM engines (< 1 %), with a decreasing number of engine suppliers for rail applications, to enable the rail vehicle manufactures to develop environmentally friendly and cost effective railway solutions.
Recommendations for amended legislation
Taking into account all these aspects vehicle manufacturers along with engine manufacturers and operators, unanimously proposed amendments of Directive 26/2004.
The inventory has clearly shown that rail sector’s contribution to the total emission amount counts for less than 1%. Even a complete electrification of the rail network would not lead to a significant emission reduction. However, if the IIIB limits were introduced without changes by 2012, there would be a potential risk of further modal shift from rail to road as the threat of closure of secondary lines becomes a reality.
To demonstrate the railways’ commitment to maintain its environmental friendly reputation, rolling stock manufacturers, operators and engine producers are submitting a common proposal to the European Commission’s 7th Framework Programme (FP7).
Up to five prototypes (DMUs, shunters and main line locomotives) will be equipped with IIIB compliant engines and operated in commercial service. Working together with universities and research institutes, an evaluation of the results will enable the most appropriate methods to reduce rail emissions to be determined which take all factors into consideration.
However, the results of the JRC study and the potential proposed FP7 project offer the opportunity to make appropriate railway specific amendments to 2004/26/EC Stage IIIB emission limits. The common approach adopted by the rail operators, rolling stock manufacturers, engine producers and the European Commission has the potential to deliver less pollution and more efficient rail diesel traction for the future.
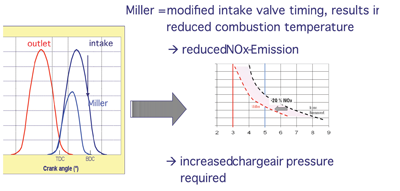
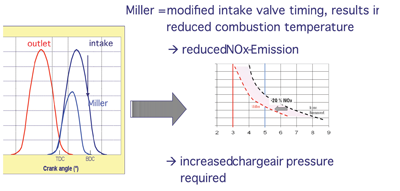
Figure 1: Functional schematic of Miller cycle
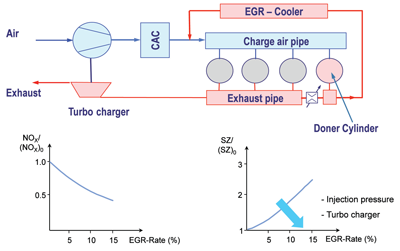
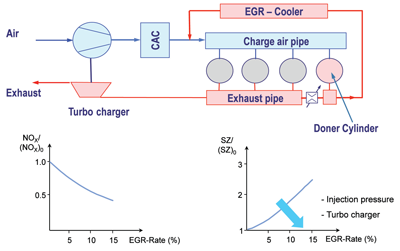
Figure 2: Functional schematic of EGR