Asset management approach for railway track developed by TU Graz
Posted: 23 November 2016 | | No comments yet
Austria’s asset management strategy has been developed at Graz University of Technology, within the Institute of Railway Engineering and Transport Economy, in close cooperation with the Austrian Federal Railways (ÖBB). Here Matthias Landgraf and Markus Enzi from the Institute present an approach that enables the holistic asset management of track, combining a strategic top-down approach that deals with the average behaviour of track, as well as a bottom-up approach based on in-depth condition evaluations.
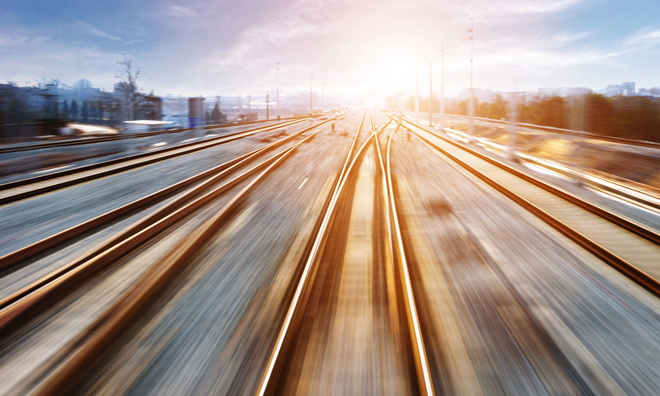
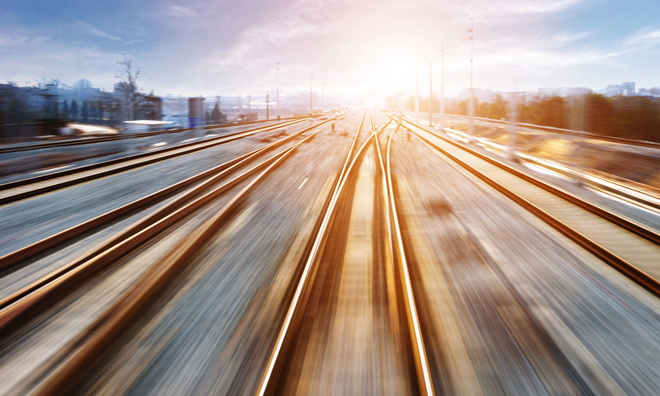
The Institute’s vast experience and research in the field of life-cycle-cost calculations1 has enabled a balance between economic and technical evaluations. The precondition for economic evaluations is an in-depth knowledge of both maintenance demands and the track’s remaining service life. We propose an approach combining two procedures:
- A top-down process for strategic planning of maintenance and renewal demands, based upon the average track behaviour for specific boundary conditions
- A bottom-up process allowing for a sophisticated track measurement evaluation that describes the component-specific track condition and its future maintenance needs.
As a crucial precondition, any asset management of track requires accurate data management. Since 2003 a data warehouse2 has been established at Graz University of Technology in close cooperation with ÖBB. Currently this data warehouse contains track information regarding more than 4,000 track kilometres of Austria’s main network. Every 5m of track, a cross-section is assigned its corresponding track information. The data warehouse currently provides linked information concerning three main points:
- General asset information (type and age of sleepers and rails, traffic volume and executed maintenance actions)
- A complete history of measurement signals since 2001, as provided by the Austrian track recording car, including calculated deterioration functions
- Evaluations from the ground-penetrating radar.
Strategic renewal demands
The estimation of strategic renewal demands can be performed using so-called standard elements that include necessary maintenance actions across the whole life span, as well as the service life of track3. The data was obtained by statistical analyses and historical experience of different combinations of boundary conditions, such as steel grade, rail profile, sleeper type, radii, traffic loads and line speed. Hence, there are over 100 different types of standard elements describing the average maintenance demands as well as the estimated service life. This information is combined within the data warehouse at every cross section. Consequently, this approach allows for a determination of the strategic remaining service life for the whole network. Figure 1 shows this information, including a distinction between wooden or concrete sleepers as well as with or without under sleeper pads4 (USP). On the one hand it is obvious that, in a network-wide view, the predominant type of superstructure is concrete sleepers without USP (64%). On the other hand, the figure shows that track sections approaching or already exceeding the estimated service life are equally distributed between concrete and wooden sleepers.
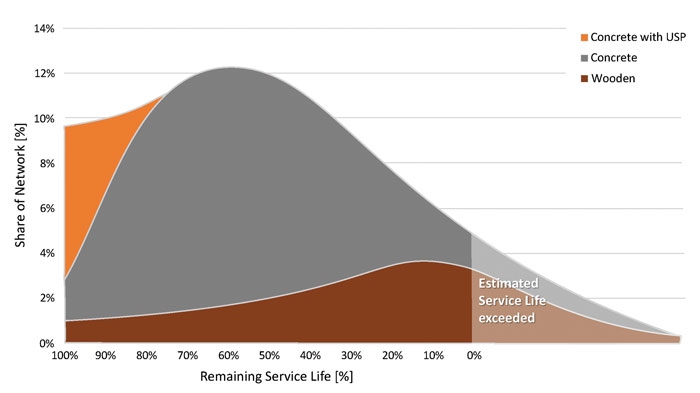
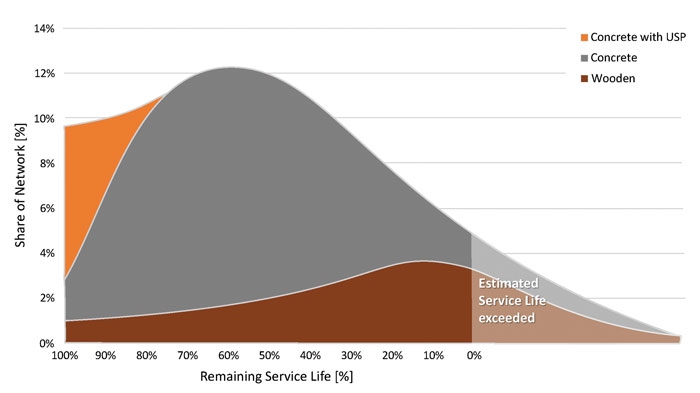
Figure 1: Estimation of remaining service life of track sections based on standard elements.
This approach can be used for estimating maintenance and renewal budgets for decades to come. These standard elements – for the whole life-cycle – include the needs and costs of maintenance. They allow for life-cycle based decisions, such as an evaluation of whether innovative components pose an economically viable solution. Since the standard element approach does not rely upon track measurements, this approach can also be applied on smaller networks where infrastructure managers do not regularly execute track measurements.
Nonetheless, standard elements only represent the average network-wide behaviour for specific boundary conditions. This means that the behaviour on specific track sections can vary significantly. Hence, a top-down approach is only applicable to a certain level of detail in terms of track section length.
Component-specific condition evaluation
The main questions for an asset manager include: “What is the main reason for track renewal?” and “What is the remaining service life of track?” Up to now it was not easy to answer these questions since it requires an identification of those components limiting the service life and a separate evaluation of their conditions. Technically, the economic service life is mainly influenced by the condition of sleepers, ballast, and substructure, since rail exchange can be executed at a much lower cost level. For superstructure with wooden sleepers, their condition is the limiting factor. Superstructures equipped with concrete sleepers will reach the end of their service life due to ballast deterioration. For concrete sleepers equipped with USP, much longer service lives can be achieved but ballast condition remains the limiting factor. In addition to these two components, substructure condition is also a crucial factor1. The life-cycle costs of track can be up to seven times higher due to insufficient substructure conditions, while service life can be as low as only half. Therefore, sleepers, ballast and substructure are the three types of components dealt with in the present paper.
For decades different types of track signals have been measured by track recording cars. Without sophisticated data management they can only be used to determine whether a value exceeds the intervention limit5. This reactive approach identifies the necessity of maintenance actions. Recently, railway infrastructure managers have been collecting and storing the data in order to monitor the track’s behaviour over time. Consequently, this preventive approach allows for a prediction when a maintenance action is presumably necessary. We want to go further by establishing a pro-active approach to answer the question as to which maintenance or renewal action should be executed. This requires a component-specific condition evaluation.
In order to establish such an evaluation, we use well-known measurement signals as well as innovative figures calculated from raw measurement data. Currently the standard deviation of vertical alignment is used to describe track quality, especially regarding ballast and substructure condition. While this type of analysis provides crucial information about whether a tamping action is necessary, the condition of the specific components as well as their remaining service life remains unknown. A bottom-up approach as shown in Figure 2 provides an in-depth assessment of track using a variety of measurement signals and an aggregated component-specific evaluation. Since the approach is based upon well positioned measurement signals, it is valid for monitoring specific track sections as well as whole networks.
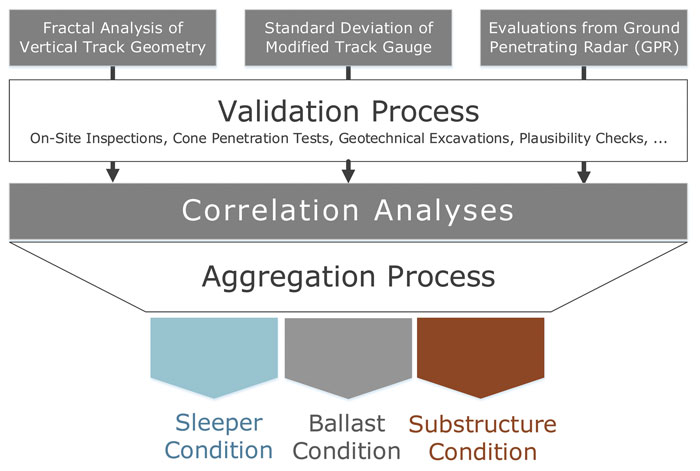
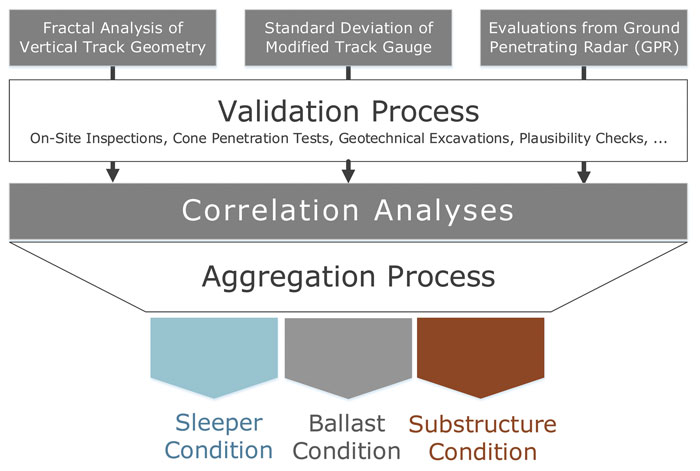
Figure 2: Establishing a component-specific condition evaluation
based on measurement signals
Innovative analyses of several measurement signals form a sound basis to grasp their characteristics, allowing for a component specific condition evaluation of railway track. Apart from exploring the behaviour of commonly used measurement signals, emphasis was placed on establishing sophisticated quality values. Two main quality values have been established to describe the component-specific asset condition:
- The standard deviation of modified track gauge6,7 describing the condition of fastenings, rail pads and sleepers
- A fractal analysis of vertical track geometry7-10 describing the condition of ballast, formation layer and subsoil.
The standard deviation of modified track gauge grasps the short-waved irregularities within the signal of track gauge, since longer wavelengths are highly influenced by gauge widening in curves. This short-waved noise describes the force interaction between fastenings and sleepers. In the case of concrete sleepers, a decreasing value of standard deviation of modified track gauge shows the necessity of a rail pad exchange. For wooden sleepers, a decreasing value indicates a degradation of the force transmission between screws and sleepers, limiting their service life.
The second quality value is a fractal analysis of vertical track geometry. This methodology is based on the fact that vertical track geometry can be considered a sum of harmonic irregularities with different wavelengths and amplitudes. Mean values and standard deviation focus only on the amplitude of defects within the alignment. By neglecting the wavelength, the characteristic of a failure remains unknown. Fractal analysis of vertical track geometry describes the characteristic of irregularities in vertical alignment by delivering expressive quality figures7,10. The so-called Modified Divider Length Method9 provides the ability to split vertical deflections into their different ranges of wavelengths, leading to separate evaluation of the conditions of ballast and substructure.
As a third main part of input data, evaluations from Ground Penetrating Radar (GPR) are included. This geophysical measurement allows for an examination of the ballast bed and the substructure. The ÖBB have been evaluating 1,400km using GPR. The applied system delivers information on Ballast Fouling, Ballast Humidity, Ballast Undulation, Interlayer Humidity, Interlayer Undulation, and Clay Fouling.
For all these evaluation methodologies, a profound validation process has been executed combining two approaches: Firstly, recorded maintenance actions and track renewals have been compared to the behaviour of fractal analysis over time, the standard deviation of modified track gauge and evaluations from GPR. Fractal analysis, for example, showed a significant improvement after ballast cleaning (mid-waved dimension) and substructure rehabilitation (long-waved dimension), respectively. Secondly, it was possible to validate these analyses to the in-situ behaviour of track. In doing so, track inspections have been carried out for visual evaluation. Moreover, soil mechanical approaches like cone penetration tests (CPT) or in-situ excavations have been executed during the validation process11,12. Finally, a statistical correlation analysis between the different methodologies has been executed. As a result, it can be stated that these evaluation methodologies allow for a description of the component-specific condition of track.
An aggregation of the different quality values is executed in order to obtain a single quality figure for sleeper, ballast, and sub structure. The weighting factors12 are established in order to calculate one comprehensive quality figure for each component. These figures are calculated as a percentage, with 100% stating the best possible condition of the component. Due to installation tolerances, a perfect condition is only of a theoretical nature, so a newlybuilt track shows values of 90-95%. The distribution results in a left-skewed Poisson distribution typical for condition evaluations.
Measurement data aided asset management
These component-specific figures yield one main question: What is the critical quality for each component? In order to answer this question we examined approximately 50km of track renewal executed between 2012 and 2015. Consequently, it was possible to determine the critical quality of the specific components that lead to a wear-induced component. This identifies track sections with one or more components reaching the end of service life. The left side of Figure 3 shows all specific cross sections of the data warehouse as dots, according to their sleeper and ballast condition, considering them directly responsible for track renewal. A poor condition of substructure is added within the third dimension by colouring the dots brown. The left side of Figure 3 is divided into four areas:
- Area I describes track sections where the sleeper condition has already exceeded the critical condition
- Area II shows the track sections without any problems concerning the component’s condition
- Area III displays sections where the ballast condition has already exceeded the critical condition
- Area IV illustrates track sections dealing with a poor condition of both sleepers and ballast.
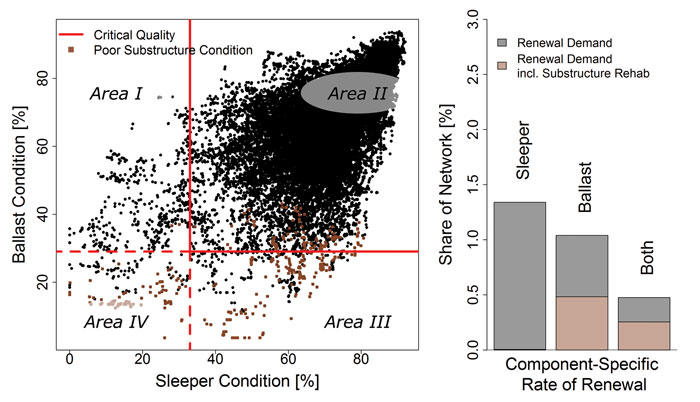
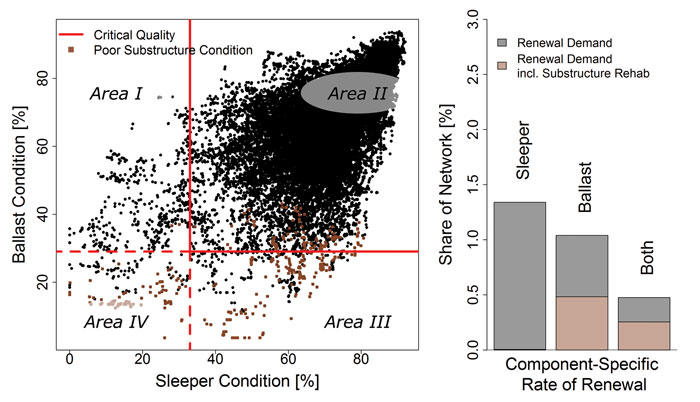
Figure 3: Renewal demand derived from component-specific
condition evaluation
Additionally, as a third dimension, the brown dots highlight sections where the substructure condition is poor. Consequently, a component-specific renewal demand can be established for the whole network. As shown on the right hand side of Figure 3, the majority of track sections exhibit either a poor sleeper or a poor ballast condition. This mirrors the aforementioned fact that a superstructure with wooden sleepers has to be renewed due to sleeper condition, while the service life of superstructures with concrete sleepers depends on the condition of ballast. It can also be shown that poor substructure condition only appears with poor ballast condition. This appears logical, since these two components interact very strongly.
This knowledge enables the specific component of track to be identified that will initiate the end of service life. Consequently, the measure which has to be executed can also be determined. For this purpose especially, the necessity for substructure enhancement is essential information as they can double the costs of renewal. Generally, it can be calculated from an economic point-of-view whether a component exchange or a total track renewal10 should be executed. In some cases it may be a preferable option to execute ballast cleaning separately as a maintenance action. Within the large majority of cases, a total track renewal is the cheaper solution in terms of life-cycle costs.
Conclusion
The presented approach enables the holistic asset management of track. It combines a strategic top-down approach dealing with average behaviour of track as well as bottom-up approach based on in-depth condition evaluations. This condition evaluation only requires track geometry data from an ordinary measurement car. Additionally, evaluations from ground penetrating radar were included in order to increase the level of detail. The methodology describes sleeper, ballast and substructure condition separately, i.e. the components causing the majority of track renewals. Hence, this methodology can be an essential element in pro-active asset management. Budget preparation as well as distribution among regional divisions can be sophisticated by using this condition-based approach.
References
- Veit P. (2007). Track Quality – Luxus or Necessity? RTR Special, pp. 1-5
- Holzfeind J., Hummitzsch R. (2008) Quality Behaviour of Track. ZEV Rail Glasers Annalen 132, pp. 212-224
- Veit P. (2002) Infrastructure Maintenance Strategies, Rail International, no. 6, pp. 2-10
- Marschnig S., Veit P. (2011) Making a case for under-sleeper pads; International Railway Journal, no. 5, pp. 25-27
- CEN/TC 256, 2005. Railway applications- Track geometry quality EN 13848-Part 5: Geometric quality assessment, Vienna: Austrian Standards Institute
- Hansmann F., Marschnig S., Landgraf M. (2013) GleisPROPHET – next step to sustainability. Proceedings, World Congress on Railway Research
- Landgraf M. (2016) Railway Track Condition: Assessment, Aggregation, Asset Management. Full Paper, World Congress of Railway Research WCRR. Milano, Italy
- Landgraf M., Hansmann F., Marschnig S. (2014) Track Geometry and Substructure Condition – a provable Correlation? GEORAIL 2014, IFSTTAR, pp. 623-632
- Hyslip J. (2002) Fractal Analysis of Geometry Data for Railway Track Condition Assessment. PHD-Thesis, University of Massachusetts
- Landgraf M. (2015) From track data to asset management, Global Railway Review, 21, no. 2, pp. 58-61
- Landgraf M., Pinter E., Stern J. (2016) Automatisierte Zustandserfassung des Eisenbahnunterbaus mittels Georadar. Eisenbahntechnische Rundschau 6, pp. 59-63. (German only)
- Landgraf, M.; Enzi, M. (2016) Component-specific condition evaluation of railway track. ZEV Glas. Ann.
140 (10), pp. 424-431. (German only)
Biography
Dr. Matthias Landgraf is a graduate of Graz University of Technology (TU Graz) where he composed his PHD-Thesis on asset management within railway infra structure. Since 2011 Matthias has been a Scientific Research Associate at the Institute of Railway Engineering and Transport Economy (part of TU Graz). He focuses on big data in railways allowing for efficient asset management in railway infrustructure, considering the costs of the whole life cycle.