Tunnelling for and into the future of European railways
Posted: 17 September 2010 | | No comments yet
The remarkable volume of tunnelling on a worldwide basis during the last two or three decades (see Figure 1) is not defined by the demands to improve the railway networks in many countries. Stimulation in this direction is especially given by the generally highly ranked socio-political requirement of improved mobility – both for persons and goods – achieving at the same time a better environmental balance.
The remarkable volume of tunnelling on a worldwide basis during the last two or three decades (see Figure 1) is not defined by the demands to improve the railway networks in many countries. Stimulation in this direction is especially given by the generally highly ranked socio-political requirement of improved mobility – both for persons and goods – achieving at the same time a better environmental balance.
The remarkable volume of tunnelling on a worldwide basis during the last two or three decades (see Figure 1) is not defined by the demands to improve the railway networks in many countries. Stimulation in this direction is especially given by the generally highly ranked socio-political requirement of improved mobility – both for persons and goods – achieving at the same time a better environmental balance.
Some extremely long tunnel projects recently inaugurated or still under construction or under planning, listed in Table 1, are amongst the most prominent and outstanding single projects. Their locations are shown by the maps in Figures 2 and 3.
Name | Country | Length | Planned inauguration |
Gotthard Base Tunnel | CH | 57km | 2017 |
Loetschberg Base Tunnel | CH | 37km | 2007 |
Ceneri Base Tunnel | CH | 15km | 2019 |
Brenner Base Tunnel | A/I | 55km | 2018 |
Mont d’Albin | F/I | 53km | 2020 |
Strait of Tatars | RUS | 12km | Unknown |
Bering Strait | RUS/USA | 97km | Unknown |
Strait of Gibraltar | E/MA | 35km | 2025 |
Koralm | A | 33km | 2013 |
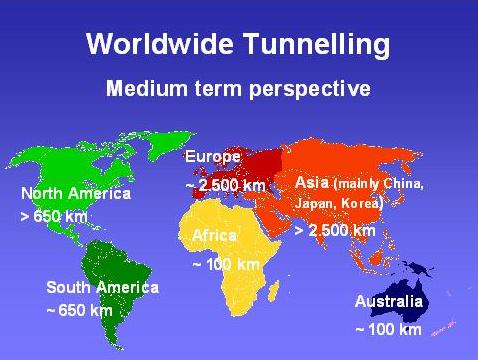
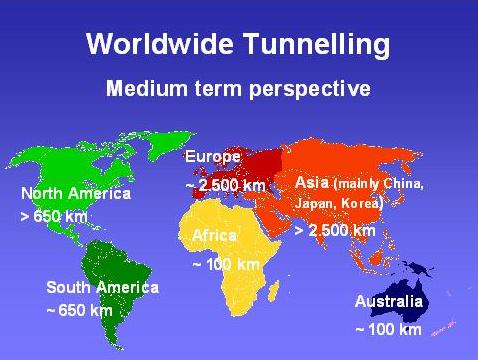
Figure 1 Expected volume of worldwide tunnelling during the next 10-15 years1
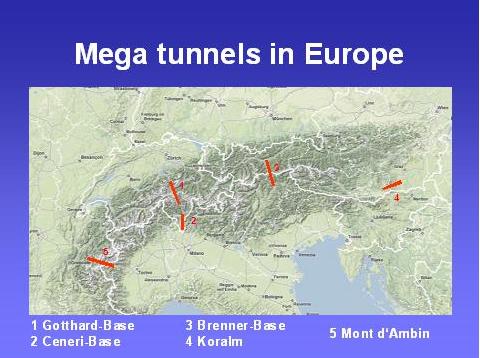
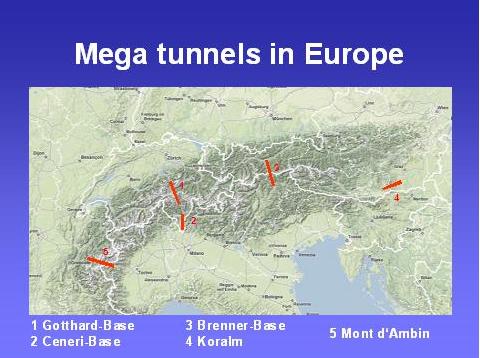
Figure 2 Extremely long railway tunnels in Europe under construction or design
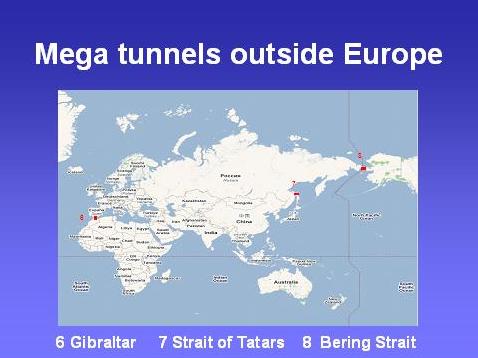
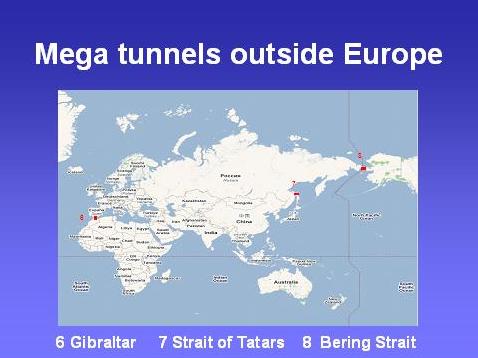
Figure 3 Extremely long railway tunnels outside Europe under construction or design
In Japan and Europe, railway traffic has experienced a renaissance since the 1970s and 1980s. This holds primarily for high-speed passenger railway lines, offering speeds between 250 and 350km/h. In Europe, France, and then some time later Germany, started to construct and operate those lines, followed by Italy, Spain and the UK. Even Austria and Switzerland are making tremendous efforts to accelerate their railway connections, despite their extremely difficult topography. After the opening of the Iron Curtain in 1989/1990, central European countries were involved in the planning of the Trans-European Railway Network (TEN-Rail), as shown in Figure 4. Here, details are given concerning the design speed for the various sections, and it shows that high-speed lines > 250km/h are concentrated in France, Germany, Italy and Spain. More detailed pictures of the situation in France, Germany and Spain can be found in Figures 5, 6 and 7. Those very fast railway links require an alignment which is as straight and flat as possible. Because of this, tunnels along the lines are unavoidable. Some of the stretches consist of more than 30-40% of tunnels, and in extreme cases up to 80% (Firenze–Bologna).
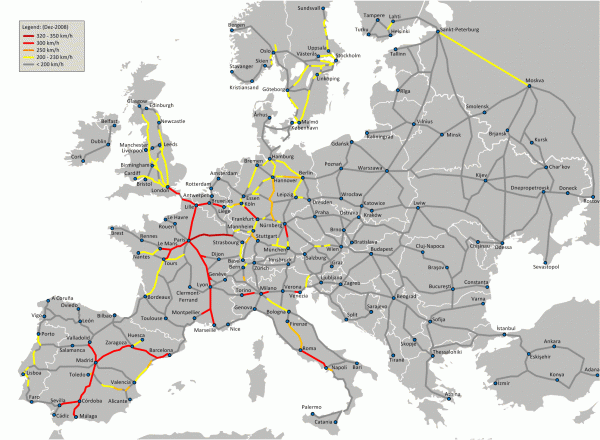
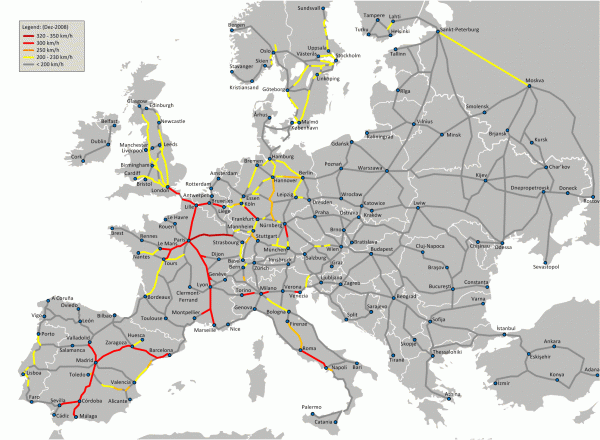
Figure 4 Trans-European Railway Network (TEN-Rail) as per 2009 Copyright: Bernese media
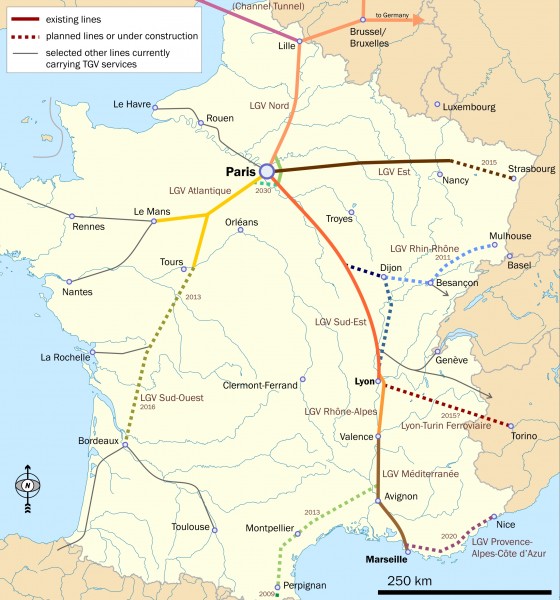
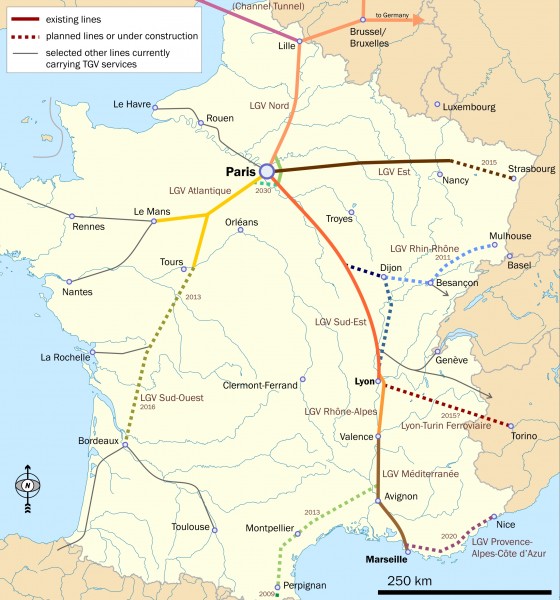
Figure 5 High-speed railway lines in France Copyright: Classical geographer
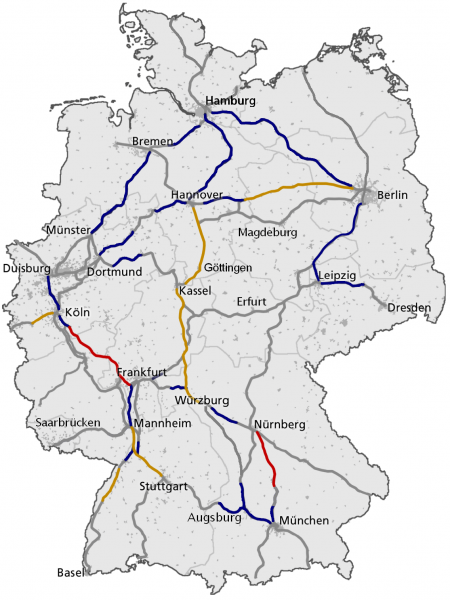
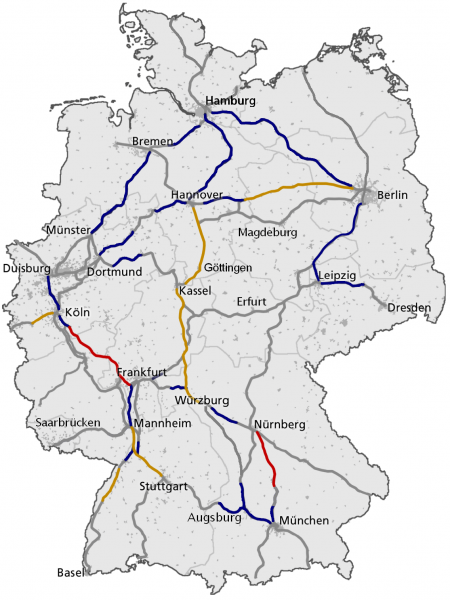
Figure 6 High-speed railway lines in Germany
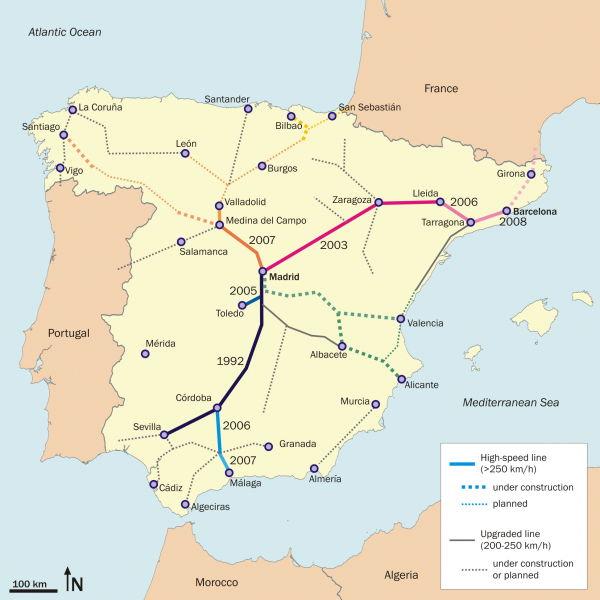
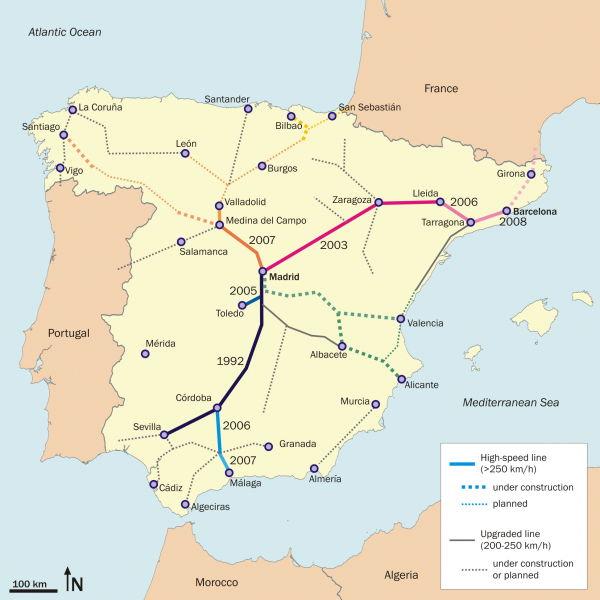
Figure 7 High-speed railway lines in Spain Copyright: MJSmit
In the following, Germany is taken as an example to describe the technological procedure to develop a railway network which meets the requirements of a modern industrial society. In Germany, there are a total of approximately 1,400km of transport tunnels currently in use (see Table 2).
Tunnel Use |
Length (km) |
Earliest date of Construction (-) |
Underground, urban and S-Bahn (rapid transit) | 658 | 1902 in Berlin |
Main line railway | 492 | 1843 route Cologne–Aachen |
Road tunnel | 247 | 1834 Tunnel Altenahr |
Total |
1397 |
In 1980, work started on the first two highspeed lines for the Deutsche Bahn AG (former Deutsche Bundesbahn) between Hannover and Würzburg as well as Mannheim and Stuttgart. Construction finished in 1991 and both lines begun ICE services the same year. Tunnels account for roughly 30-35% of the total length along these high-speed routes which, on the one hand, were selected to facilitate such high speeds but, on the other hand, for ecological reasons. Altogether, 87 twin-track tunnels with a total length of some 150km were built, with a longitudinal gradient of 12.5‰, on which freight trains can also operate. Along these two new routes, the tunnels have a clear cross section of 80m2 and an excavated area of between 110m2 and 150m2, which means they possess considerably greater cross-sections than the older twin-track railway tunnels being in service up to that point – with a clear crosssection of roughly 46m2. The clear cross-section for the new Cologne–Rhine/Main and Nuremberg Ingolstadt lines (see Figure 8 and Figure 10), which are purely designed for passenger trains with a travelling speed of up to 300km/h, is even greater with 92m2 for aerodynamic reasons. They consist of 30 tunnels with a combined length of 47km and a longitudinal gradient of 40‰, and nine tunnels with 27km length respectively.
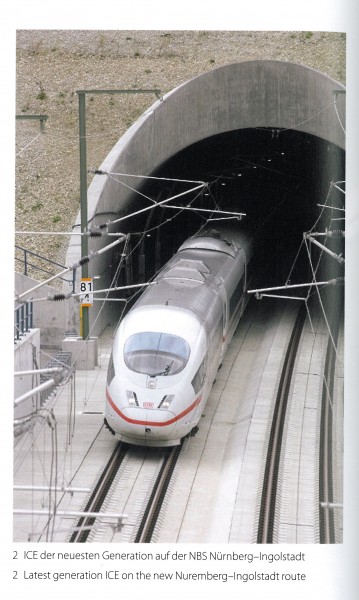
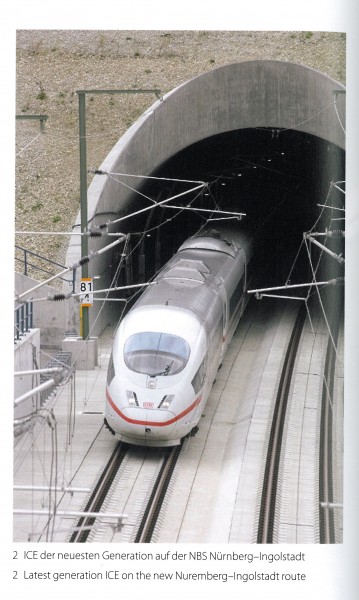
Figure 8 Latest generation of ICE trains on the new Nuremburg–Ingolstadt route3
During the last 20 years, the total length of operational transport tunnels in Germany has grown from approximately 850km to more than 1,400km. This increase is partly to do with ongoing urban rapid transit railway construction, with an annual rate of completion corresponding to roughly 1km (see Figure 9). On the other hand, as far as the railways are concerned, around 220km of highly modern tunnels have been opened since 1990, in addition to the existing network, mainly in conjunction with the high-speed lines Hannover–Würzburg, Mannheim–Stuttgart, Cologne–Rhine/Main and Munich–Nuremberg. The annual completion rate on this sector also amounted to around 11km on a long-term average. Finally, some 8-9km of additional tunnels have been opened for traffic in conjunction with federal highways since 1990.
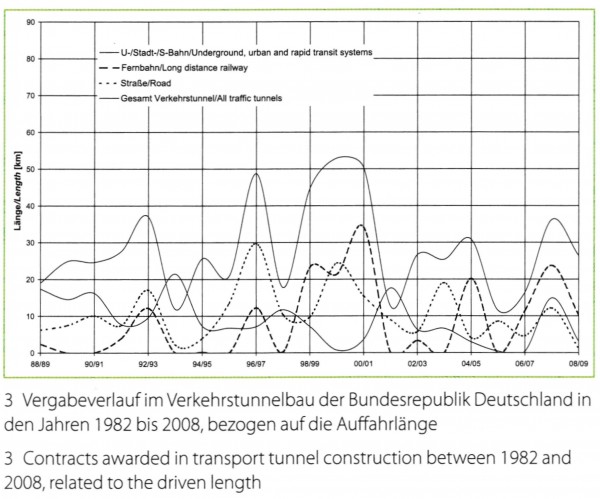
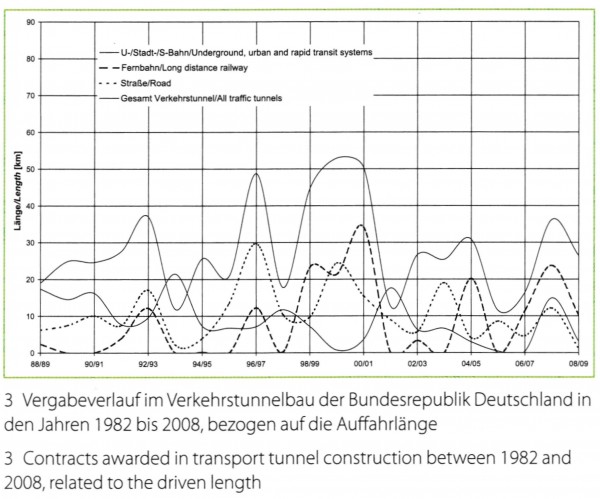
Figure 9 Contracts awarded in transport tunnel construction in Germany between 1982 and 2008, related to the driven length3
The powerful influence of main line rail tunnels can clearly be seen in Figure 9. The curve shows the placing of contracts, by and large, and follows the summation curve of the activities of the Deutsche Bundesbahn/Deutsche Bahn AG. Following a steep increase in 1982 to 1984, an equally steep drop was registered for main line tunnels during the two to three years that followed. Ultimately, at the end of the 1980s, the curve reached the zero mark, only to experience minor temporary highs during 1991/1992 as well as 1995/1996 and then again from 1998.
The tunnel volume anticipated in the near future (10-15 years) is of particular interest and results to about 400km for transport tunnels. This will largely be realised by the year 2025, according to the latest level of planning. Apart from some 40km of underground, light railway and rapid transport tunnels, the volume of practically 200km for main line tunnels and 160km for road tunnels is remarkable. The amount of main line tunnels mostly belongs to the high-speed lines Nuremberg–Erfurt and Erfurt–Leipzig/Halle as well as to the project Stuttgart 21 in combination with the highspeed line Wendlingen–Ulm, comprising a combined total length of tunnels of 116km.
At present, the new high-speed line Nuremberg–Erfurt–Leipzig/Halle4 with a total length of about 230km is under construction and planned for inauguration between 2015 and 2017. Along this route, 25 mainly twin-track tunnels with a total length of 56.4km are to be built (see Figure 11). They are designed for a maximum travelling speed of 300km/h (see also the Table in Figure 10) and undercross the low mountain range of the Thueringer Wald between Ilmenau in the south and Coburg in the north. The excavation of these tunnels is conducted by drill and blast.
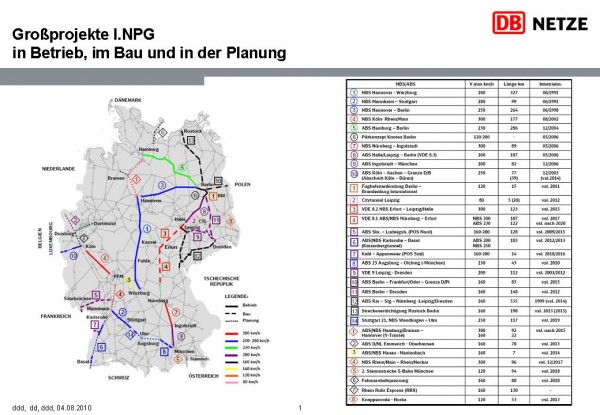
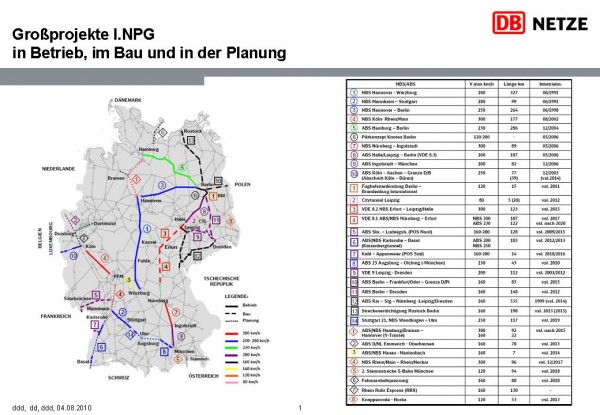
Figure 10 Major projects for railway infrastructure in Germany in operation and under construction or design Source: DB Netz AG


Figure 11a Longitudinal section of high-speed line Ebensfeld–Erfurt4 – Southern tunnel part
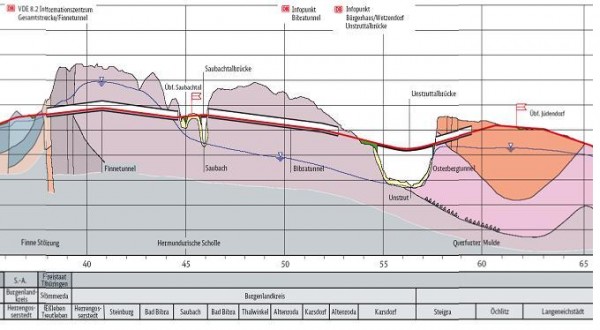
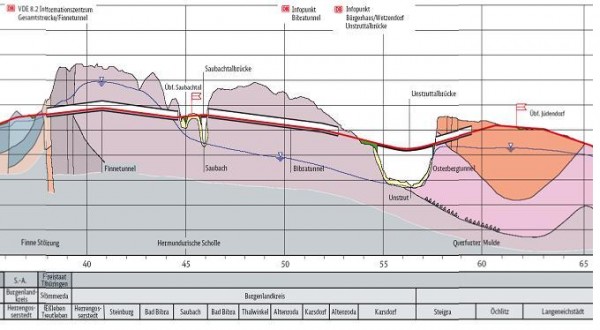
Figure 11b Longitudinal section of high-speed line Ebensfeld–Erfurt4 – Northern tunnel part
The 6.970m Finne Tunnel is part of the 123km-long northern section high-speed line Nuremberg–Erfurt and Erfurt–Leipzig/Halle. The Finne Tunnel is the only tunnel to be constructed by means of a tunnel boring machine5 (TBM) (see Figures 12, 13 and 14). The tunnel consists of two parallel tubes which are linked every 500m by maintenance and emergency cross-passages. Both tubes are driven parallel by two staggered TBMs. The shield machines possess an external diameter of 10.82m. The segment thickness amounts to 45cm – given a ring width of 2m – and the internal diameter is 9.60m. The two TBMs made by Herrenknecht AG are devised as convertible mix-shields.
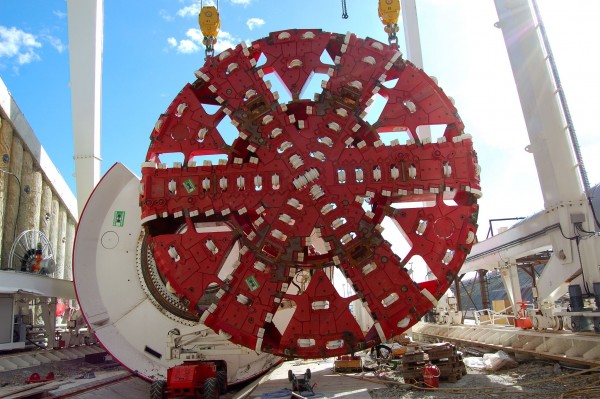
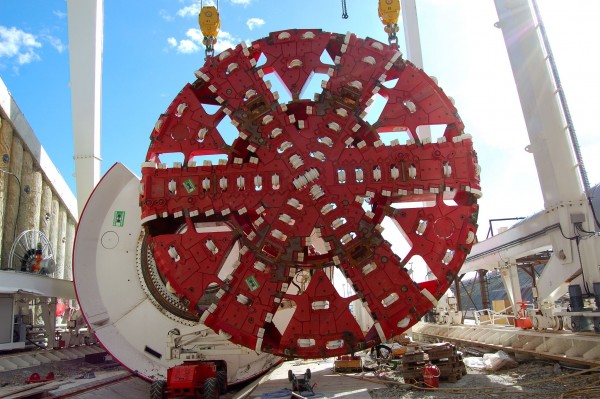
Figure 12 Cutterhead of the TBM for the Finne Tunnel5
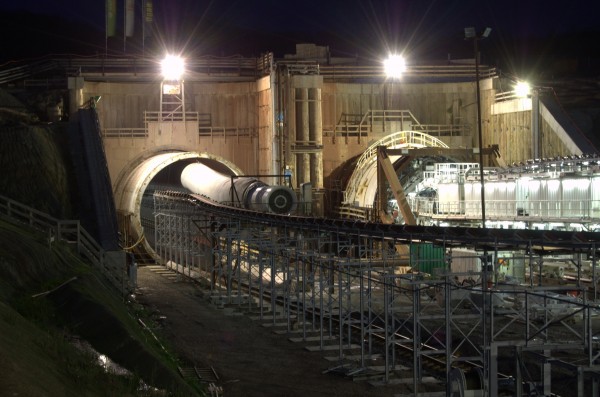
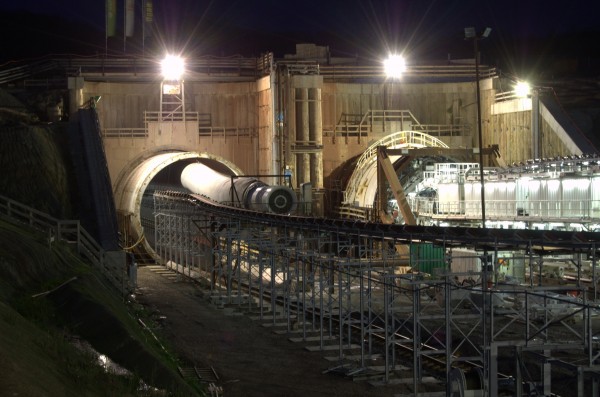
Figure 13 The two parallel bores of the Finne Tunnel5
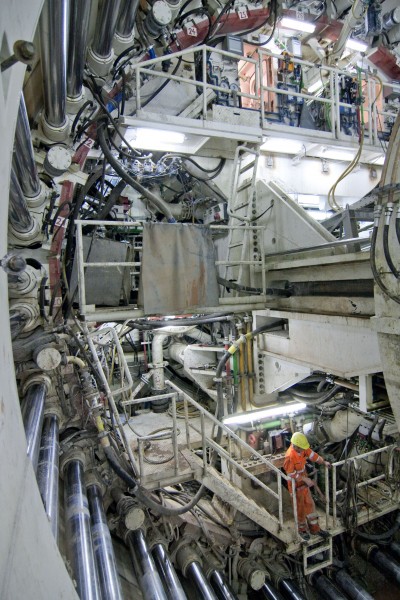
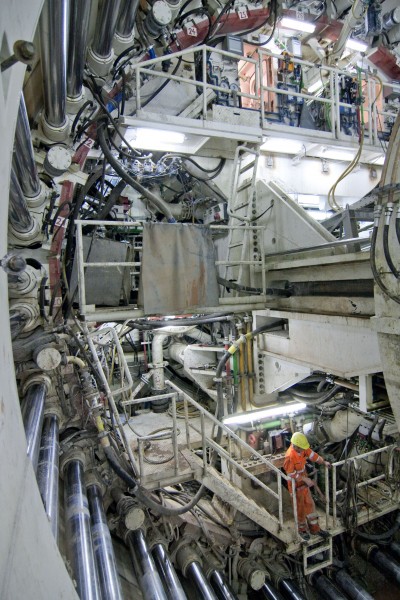
Figure 14 Back side of the TBM for the Finne Tunnel5
Over the first 1,500m of the excavation, the TBMs were operated as hydro-shields in closed mode with fluid support, then they were converted underground from closed to open mode. Towards this end, the cutterhead was adjusted to without conversion chamber. The hydro-shield components, such as locks etc. were removed, and the specific open mode components, such as belt conveyance, were installed.
Over the first 1,500m, the prevailing geology was characterised by alternating beds of clays, schluff stones and sandstones, which were completely disintegrated at the first and second Finne fault zones. In addition, middle and lower keuper was found over the first 250m of the tunnel. As the groundwater is located up to 60m above the tunnel crown from tunnel metre 0 to 1,500 and the inflow of groundwater was very high, these areas have to be tackled by means of a hydro-shield. From tunnel metre 1,500 until the end at tunnel metre 6,822, stable intermittent beds of sandstone and clay prevail, which can be driven using the open TBM mode. The prevailing groundwater here is also present up to 60m above the tunnel crown and is lowered from tunnel metre 1,500 to 6,000 by means of a drainage system consisting of drilled wells. Over the final 800m the water level is located below the tunnel axis. The end of the excavation work is scheduled for early summer 2010.
Closing
Safe and efficient modern railway tunnels along high-speed lines significantly contribute to accelerated passenger transport, and also assist in protecting the environment by helping to reduce air traffic. The European, Asian and North American industrial countries make use of this technology, flanked by the international railway industry.
References
1. Haack, A.: Building for the future; World Tunnelling 10 (2008) 7/8, p. 28-29.
2. Haack, A.: Tunnelling for European highspeed railways; Global Railway Review 12 (2006) 4, p. 68-72.
3. Haack, A.: Statistics, Analysis and Outlook of tunnels in Germany; Underground Construction Germany, published to mark the STUVA-Conference ’09 in Hamburg, Germany, 2010, Dec. 1 to 3, 2009 by the STUVA – Studiengesellschaft für unterirdische Verkehrsanlagen e. V., Cologne and the DAUB – Deutscher Ausschuss für unterirdisches Bauen e. V., Cologne, p. 87-93.
4. Feldwisch, W./ Drescher, O./ Flügel, M./ Lies, S.: Die Tunnel der Neu- und Ausbaustrecke Nürnberg – Erfurt – Leipzig/Halle; Eisenbahntechnische Rundschau (2010) 4, p. 186-190.
5. Azer, H./ Engel, B. and others: Railway Tunnels in Germany; Underground Construction Germany, published to mark the STUVAConference ’09 in Hamburg, Germany, 2010, Dec. 1 to 3, 2009 by the STUVA – Studiengesellschaft für unterirdische Verkehrsanlagen e. V., Cologne and the DAUB – Deutscher Ausschuss für unterirdisches Bauen e. V., Cologne, p. 124-149.
About the Author
Prof. Dr.-Ing. Alfred Haack
Prof. Dr.-Ing. Alfred Haack is a civil engineer and studied at the Technical Universities of Hannover and Berlin. He obtained his PHD degree for research on fire protecting during tunnelling in 1971. He has been working with STUVA for more than 40 years in widely varying fields of tunnelling and micro tunnelling such as drainage methods, waterproofing techniques, various lining systems, occupational health and safety, fire prevention and fire fighting. Since 1995 until 2007 he has been an Executive Board Member of STUVA and, since 1996, an honorary professor in the field of civil engineering at the Technical University of Braunschweig. Between 1995 and 2004, Prof. Haack was member of the Executive Council of ITA – International Tunnelling and Underground Space Association and between 1998 and 2001 the ITA’s President. Since 2008 he is retired and works as a free consultant for STUVA.